Reconstructing the past: the evolution of landscapes and reorganization of rivers under ancient ice
- Miquéla Thornton
- Jan 11, 2022
- 7 min read
New research offers us a window into landscapes and riverscapes prior to the Last Glacial Maximum, documenting their evolution and providing us a better understanding of ice-age legacies and current dynamics in the era of modern climate change.
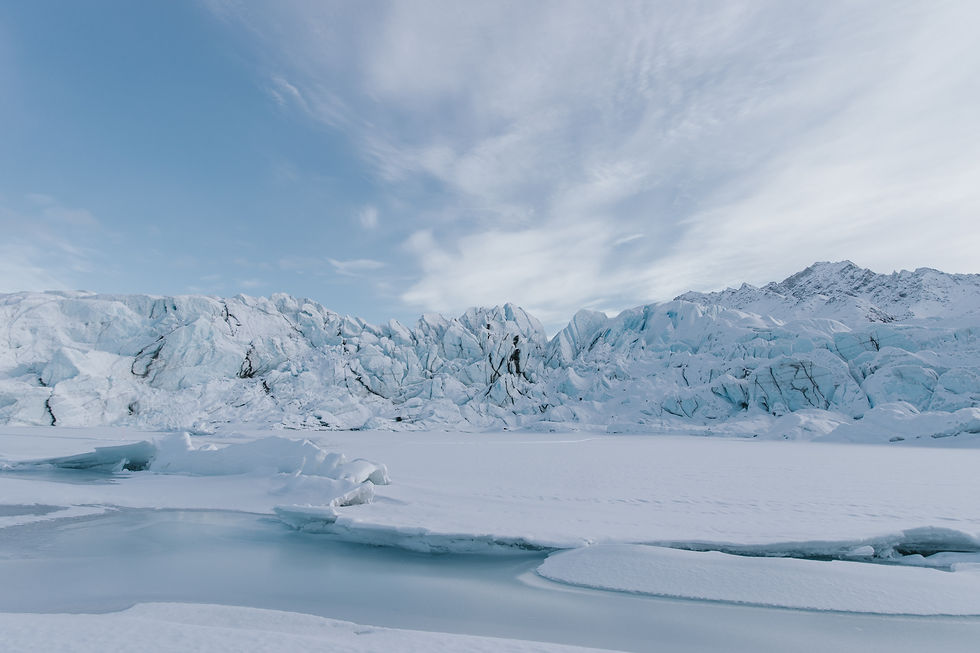
Approximately 2,600,000 to 11,700 years ago, the majority of the Earth’s surface was a polar wasteland. At the exodus of the Last Glacial Maximum, about 27,000 years ago, the Northern Hemisphere was a tundra, with the land blanketed in cloaks of ice. The last Maximum marks the span of years where ice sheets, masses of glacial land ice, reached their individual apices intercontinentally, after an era of global cooling catalyzed by the Earth’s varying orbit around the sun. The largest of them all, the Laurentide Ice Sheet (LIS), engulfed the Arctic and a third of North America. In some places the sheets reached as high as four kilometers. Land untouched by glaciation was laden with tundra vegetation, roamed by ice-age animals: mammoths, reindeer, and arctic hare.
Collectively, this epoch is known as the Quaternary, comprising the Pleistocene and Holocene. During which, the LIS expanded and retreated as the Earth’s climate moved between warm periods and ice ages, shaping the North American continent—especially the modern American Midwest—modifying the landscape, depositing agriculturally-productive sediments, carving out the basins of the Great Lakes, and sculpting the path of the Mississippi River. During these advances and withdraws, the Laurentide deposits buried hidden topography of river gorges, prehistoric valleys, drainage divides, and glacially scoured bedrock: scratches left in the surface from glacial flow through a process known as abrasion. These buried natural artifacts serve as a record of the past 2.6 million years of landscape evolution. Understanding these dynamics and thus, how the landscape developed, is vital to understanding long-term behavior of the land. These relationships are usually studied through reconstructions: time traveling telescopes into the past. Thus, new research published in the November 2021 issue of Science Advances does just that: reconstructs the past. Through an analysis of landscape evolution under the southern Laurentide Ice Sheet, the research suggests subglacial erosion was sufficient to lower the southern LIS into warmer environments, thus enhancing ablation (ice and snow removal) and reducing the extent of ice sheets over time.
The researchers, Shawn Naylor, Andrew Wickert, Douglas Edmonds, and Brain Yanites came to their conclusion by documenting the landscape beneath the southern Laurentide Ice Sheet since the end of the Piloscene, occurring 2.6 million years ago. This era represents the final stages of global cooling leading up to the Quaternary ice ages. Through digital elevation models, or DEMs, they reconstructed several features of the icy region's paleotopography including preglacial surface and modern bedrock topography, drainage networks and river profiles, to create a comprehensive view of the ancient region, and reveal both patterns and extent of the glacially-forced river reorganization. They also employed a process known as ice sheet modeling which integrates observational data with dynamical models, by creating computational models to calculate how ice sheets move in response to gravity and reveal past shapes and flows.
To quote the paper, “combining this [reconstruction models] with maps of the bedrock and modern land surface illuminates the power of ice in reshaping topography.”
Studying subglacial landscapes after recent ice-sheet retreat, such as the reorganization of rivers after ice sheet changes, provide a window into ice sheet dynamics and interactions with evolving subglacial topography. Generally, the value of landscape evolution theories can be understood as critical in comprehending interactions between climate, tectonic, and surface processes. Because the geologic processes of our world are interconnected rather than isolated events, they must be understood through their relationships. By understanding landscapes molded by the LIS in particular, researchers are able to accurately reconstruct its history and examine the long-term behavior of ice sheet models, as well as the feedback between ice and landscape dynamics. According to the study, theoretically these ancient feedbacks may have caused the mid-Pleistocene transition, generated marine ice sheet instability, and resulted in the prominent glacial advances of the Early Pleistocene era. Including the dramatic climate swings of the Pleistocene into models is essential in understanding the effects of present-day climate and environmental change on modern glacial and subglacial topography.
Evidence for at least 10 different advances and retreats of the LIS is found in existing glacial deposits and modeling. Due to the isolation of these events from existing models, the cumulative impacts on the landscapes are massively unknown; a fact accentuated by the lack of reconstruction of end-Pliocene topography, a large factor in the trajectory and pace of landscape evolution. Without a map of bedrock erosion, understanding the interplay between the LIS and North American landscape is onerous. By directly accessing the amalgamated effects of LIS advances and retreats on erosion and deposition, the researchers were able to 1) provide a necessary starting point to subsequent testing of feedbacks between landscape evolution and ice-sheet dynamics and 2) provide the “first glimpse” of a quantitatively reconstructed topographic surface of North America prior to glaciation.
However, this type of research isn’t limited to the Midwestern landscape, and is also applicable to river systems across the North American continent. Glacial isostatic adjustment (GIA), is the ongoing movement of land once encumbered by ice-age glaciers. Ice sheets and their resultant GIA rearranged river systems over the last glacial cycle. Thus riverine threads that tied the ice sheets were stretched, severed, resized, and restructured. This reorganization sometimes even delivered enough meltwater to the oceans to influence global climate.
To reconstruct this, glaciologist Andrew Wickert of the University of Minnesota, created a method to compute past flow paths, drainage basin geometries, and river discharges (as utilized in the aforementioned study) by combining various models of past ice sheets, glacial isostatic adjustment, and climate. The study, published in 2016 by Earth System Dynamics, resulted in synthetic paleo-hydrographs and drainage basin maps from the Last Glacial Maximum across North America, comprising of nine major drainage basins: the Mississippi, Rio Grande, Columbia, Mackenzie, Hudson Bay, Saint Laurence, Hudson, and Susquehanna/Chesapeake Bay, as illustrated on the past and present maps below, outlined in black.

Along with the objective of providing a general computation method, Wickert aimed to provide a complementary framework for ice-sheet reconstructions. The success of evolutionary ice sheet reconstruction depends heavily on the abundance and quality of observational data available. Thus, Wickert based the work off five published reconstructions of North American ice sheets. He then compared them to a number of different forms of observational evidence from river deposits to glacial moraine histories, to isotopic records to mineral markers. To quote the paper, “The work presented here is a necessary starting point for future studies that investigate the interactions between deglacial surface-water hydrology and other components of the integrated Earth system.” Moreover, as the paper articulates, this work can be used to reconstruct archeological areas of interest, and as the collection of records from the North American continent and continental margin become increasingly more complete, new possibilities of whole ice sheet isotopic studies emerge. While this work is essential for understanding ice sheet geometry and dynamics post-glaciation, the paper highlights the significance of the North American deglacial drainage basins in their own right: an “ice-age legacy imprinted on major rivers of the continent.”

Another benefit of this work is predicting the results of climate change by using models to assess the stability of ice sheets past and present and forecast how these changes, such as melting, will contribute to sea level rise. As said by researcher Henry Patton in an interview with Science Direct on his team’s latest study reconstructing the Eurasian ice sheet, “One of the major questions facing us today is how the present ice sheets in Greenland and Antarctica will react to climate change. Simply put, the more we understand of the mechanisms that drove ice sheets to collapse in the past, the better we will be able to predict what will happen in the future.”
Cycles of warming and cooling are normal. Afterall, the last glaciation of Earth started 37,000 years ago when the planet’s climate began to drastically cool, and repeated consistently over the last million years or so: every 100,000 years, 90,000 years of ice age are followed by 10,000 years of an interglacial warm period. However, due to present global warming, our current warm period is out of the ordinary, a fact illustrated by the Laurentide Ice Sheet.
The LIS began to disappear during the genesis of the last warming period and by 8,000 years ago the Laurentide Ice Sheet was constricted to the area that is now Quebec and Labrador, decreasing from a casing that covered much of the world to a mass roughly the size of Greenland’s ice sheet. Now, what’s left of the Laurentide Ice Sheet is about the size of Delaware: a glacier called the Barnes Ice Cap located on Buffins Island in Canada. Once sustained by the present interglacial climate and its own topography, the ice cap is expected to vanish in the next 300 years after 2,000 years of stability. As projected by a 2017 study, this anticipated melting is due to an unusually warm 21st century Arctic, the product of unparalleled rise in greenhouse gases. Thus, while melting is normal and a part of natural Earth cycles, scientists note the glaciers are melting at an alarming rate. As explained by Daniel Fagre, a research scientist from the U.S. Geological Survey Global Change Research Program, “Things that normally happen in geologic time are happening during the span of a human lifetime.”
Thus, the next steps of many reconstruction projects is modeling the collapse of ice sheets to comprehend their climatic, geological, and oceanographic sensistives, traits likely shared by what is left of today’s glaciers as they quickly collapse. Reconstructing both collapse/formation, and landscapes prior to/after glaciation aim to answer the fundamental, overarching question of what happened to the Earth’s climate and glacial topography before the age of humans, and what is to come after.
Comments